The most powerful use of SAXS is in the real time study of biological macromolecules undergoing structural changes in response to a variety of conditions such as denaturant / renaturant concentrations, ionic strength, ligand binding, enzyme-substrate interactions etc…. Different types of mixer- technologies give access to different time regimes (Fig. 1). These mixers have traditionally been used in combination with spectroscopic techniques such as Fluorescence Resonant Energy Transfer (FRET) which yield valuable information about localized structural alterations in response to changes in the micro- environment. We have successfully combined these mixing techniques with specifically designed SAXS cameras at BioCAT. Our time-resolved instruments give us the ability to investigate global structural changes in real time and also capture intermediates that are difficult to detect using other techniques. We have three different time-resolved SAXS instruments.
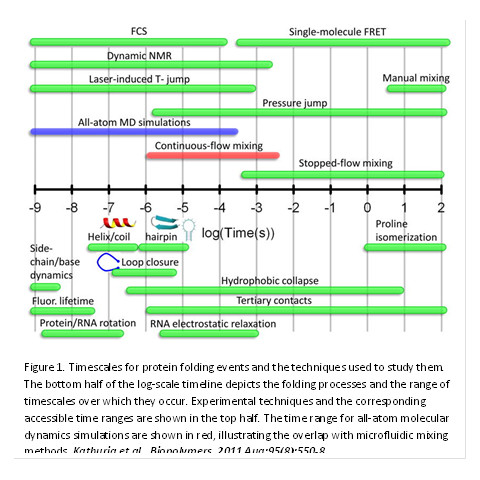
In the following video from the 2022 Everything BioSAXS 8 workshop, Dr. Jesse Hopkins discusses the current state of time resolved SAXS at BioCAT (get slides)
Stopped-Flow
We have an SFM-400 (Bio-logic science instruments) stopped-flow instrument equipped with a flow cell containing a 1 mm quartz capillary with 10µm walls specifically tailored for SAXS (Fig.2). We have achieved a dead-time of ~1 ms and can extend data collection to a few hundred milliseconds depending on the radiation sensitivity of samples. The Pilatus3 1M detector, with a 1 ms readout time affords us optimal temporal resolution. Stopped-flow SAXS is the only time-resolved instrument that operates on the main beamline optics (beam size - ~150 µm horizontal X 75 µm vertical).
Chaotic/Turbulent Flow
The main disadvantages of stopped-flow SAXS are a very high likelihood of radiation damage of the static plugs of liquid and low signal to noise ratios due to the extremely brief exposures needed for optimal temporal resolution. Continuous flow mixers are an alternative which depend on the mixing of two or more streams of fluid followed by an observation channel the distance in which corresponds to the age of the mixture. While continuous flow is an obvious strategy to circumvent radiation damage and signal to noise issues, sample consumption tends to be high. However, developmental efforts over the past few years at BioCAT have resulted in significantly smaller beams using a compound refractive lens (CRL - 20µm horizontal X 5µm vertical), which in turn has enabled us to design much smaller microfluidic chips that reduce sample consumption by orders of magnitude (10-50 mg of sample). The latest version of the mixer can be used to measure time regimes in the ~100 µs to 75 ms range. Our optimized system consisting of the CRL, a quartz mixer with ideal optical properties, efficient fluid delivery systems (Harvard syringe pumps and Teledyne ISCO HPLC pumps), and a fast readout photon counting detector (Pilatus3 1M) can be deployed every run upon request and appropriate vetting of the experimental systems, which must be initiated by contacting beam line personnel.
Laminar Flow
We have also developed a laminar flow mixer that is compatible with our CRL SAXS setup. Laminar flow mixers use hydrodynamic focusing to mix multiple streams of liquid and is able to do so at much lower flow speeds compared to turbulent / chaotic flow mixers. Sample consumption is therefore considerably lower, however, the extremely small sample profiles generated by hydrodynamic focusing result in sub-optimal signal to noise ratios therefore necessitating use of much higher concentrations. The time regimes that can be sampled using laminar flow mixers ranges from ~1 ms to 1.5 s. As with the other two time-resolved SAXS techniques, there is a particular need for preparatory rigor and is therefore important to discuss the experiments with beamline personnel well in advance.
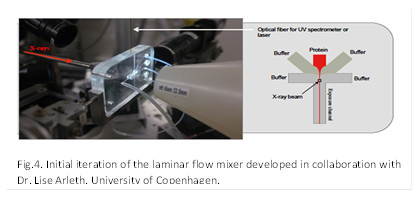
Selected Highlights
Atomistic structural ensemble refinement reveals non-native structure stabilizes a sub-millisecond folding intermediate of CheY. Shi J, Nobrega RP, Schwantes C, Kathuria SV, Bilsel O, Matthews CR, Lane TJ, Pande VS. Sci Rep. 2017 Mar 8;7:44116. doi: 10.1038/srep44116. PMID: 28272524
Microsecond barrier-limited chain collapse observed by time-resolved FRET and SAXS. Kathuria SV, Kayatekin C, Barrea R, Kondrashkina E, Graceffa R, Guo L, Nobrega RP, Chakravarthy S, Matthews CR, Irving TC, Bilsel O. J Mol Biol. 2014 May 1;426(9):1980-94. PMID: 24607691
Modulation of frustration in folding by sequence permutation. Nobrega RP, Arora K, Kathuria SV, Graceffa R, Barrea RA, Guo L, Chakravarthy S, Bilsel O, Irving TC, Brooks CL 3rd, Matthews CR. Proc Natl Acad Sci U S A. 2014 Jul 22;111(29):10562-7. PMID: 25002512
Advances in turbulent mixing techniques to study microsecond protein folding reactions. Kathuria SV, Chan A, Graceffa R, Paul Nobrega R, Robert Matthews C, Irving TC, Perot B, Bilsel O. Biopolymers. 2013 Nov;99(11):888-96. PMID: 23868289
Sub-millisecond time-resolved SAXS using a continuous-flow mixer and X-ray microbeam. Graceffa R, Nobrega RP, Barrea RA, Kathuria SV, Chakravarthy S, Bilsel O, Irving TC. J Synchrotron Radiat. 2013 Nov;20(Pt 6):820-5 PMID: 24121320
Multistage collapse of a bacterial ribozyme observed by time-resolved small- angle X-ray scattering. Roh JH, Guo L, Kilburn JD, Briber RM, Irving T, Woodson SA. J Am Chem Soc. 2010 Jul 28;132(29):10148-54. doi: 10.1021/ja103867p. PMID: 20597502