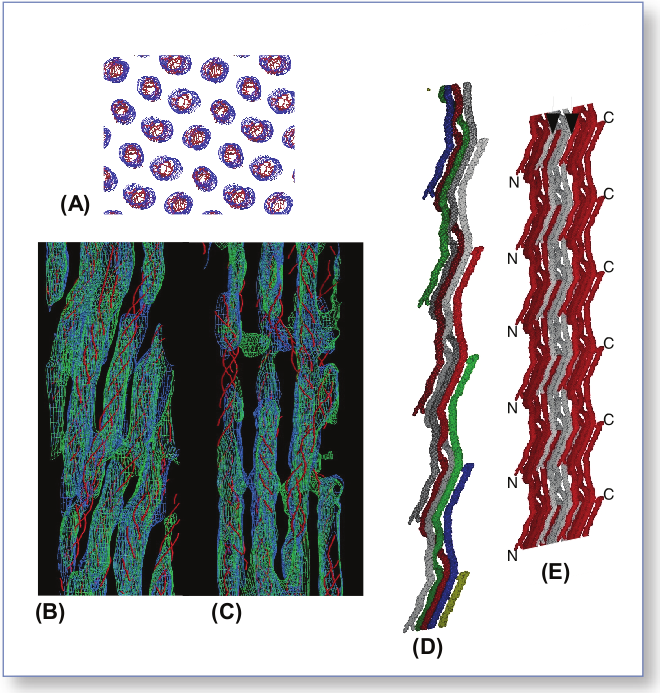
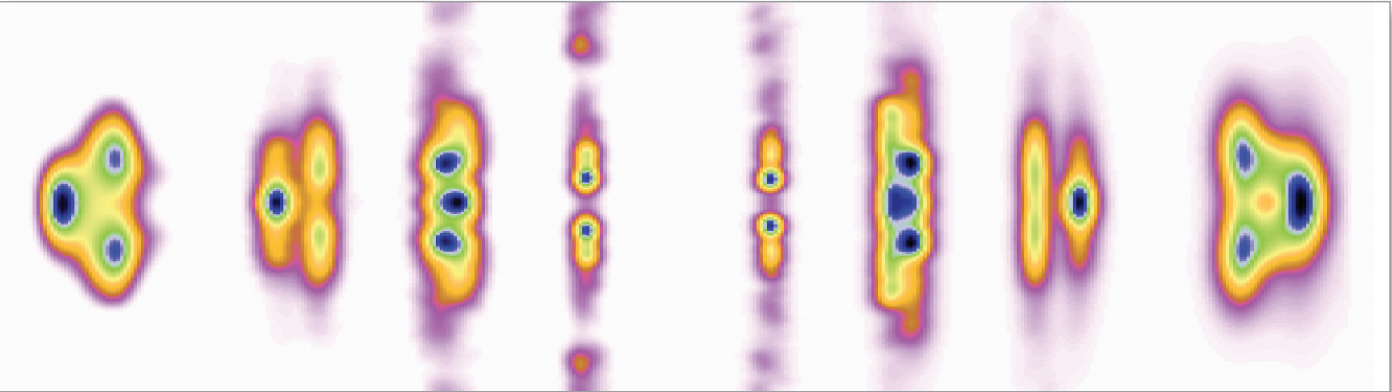
Collagens—we might take them for granted, but without them there would be no way to build tissues of the heart, skin, cornea, or bones. In much the same way that wood is used to frame a house and form a structure for the overlying construction materials, collagens are proteins used in the framing of mammalian tissues, but gaining an accurate picture of their three-dimensional structure in the body has proven more difficult. Knowing more about the structure of collagens could help biochemists improve their understanding of heart disease and cancer. Thanks to work by a research group based at the Illinois Institute of Technology and using the BioCAT 18-ID beamline at the APS, a complete structure for a collagen molecule—as it actually appears in the extracellular matrix (ECM)—is now available. This new knowledge of collagen structure provides important insights about several molecules involved in human disease.
The research group was able to determine a three-dimensional structure for a collagen (Type I from intact rat-tail tendons) as it would actually appear in the ECM. The structure is breathtakingly complex. The entire super-unit of collagen contains collagen segments packed together such that neighboring molecules, known as microfibrils, are super-twisted and then interdigitated with each other (Fig. 1). The interdigitation appears to be responsible for the super-lattice, which is constructed of quasi-hexagonally packed collagen molecules. The researchers constructed a new electron density map that, for the first time, clearly shows how all of the molecular segments, including the previously unresolved gap region, are situated (Fig. 1C). Also for the first time, the complete path of each collagen molecule can be visualized, including how the interdigitated microfibrils are arranged. And, perhaps most importantly, these data (Fig. 2) all pertain to the native structure of the collagen—how it would actually appear in living tissue.
The term “microfibril” has been used to name the basic units, or boards, of the frame created by the collagen molecules. The exact structure of the microfibril itself has been elusive—until now. By tracing the path of a single collagen molecule through several unit cells, the researchers solved this mystery. What their analysis reveals is that the microfibril is constructed of five one-dimensional, pleated collagen molecules, with a right-handed super-twist. This microfibril unit then packs with its neighbor to form the observed interdigitation. This intricate packing explains why individual microfibrils could not be isolated—it is not possible to remove them from the superstructure without destroying their own structure.
This new information about how the collagen molecules are stacked and connected makes it possible to develop hypotheses about how two molecules related to human disease—decorin and the Matrix Metallo-Proteinase (MMP)— behave. Decorin appears to be important in proper tissue construction via microfibrillar and fibrillar packing; when the gene for decorin is disrupted, fragile skin and connective tissue diseases, such as Ehlers-Danlos syndrome, can ensue. The new collagen structure provided by the research group shows sharp turns in a gap region, freeing up molecular space that could be used for decorin binding, especially on the surface of the structure. Collagenase (MMP1), which is active in the extra-cellular matrix (and, when malfunctioning, is implicated in heart disease and cancer), is thought to rely on the superstructure of the collagen molecule for normal binding and activation. Now that this three-dimensional collagen structure can be clearly envisioned, exactly what happens when MMP functioning goes awry can be postulated.
The newly published collagen structure is especially important because it is specific with respect to the microfibrillar substructure, as well as the overall superstructure, which leads to important insights into the way collagen binds other extra-cellular matrix molecules. These data will fill in several gaps for researchers studying the biology of the extracellular matrix and related tissue disease, where framing is all-important in enduring stability.
See: J.P.R.O. Orgel, T.C. Irving, A. Miller, T.J. Wess, “Microfibrillar Structure of Type I Collagen in situ,” PNAS 103 9001 (2006). DOI:10.1073pnas.0502718103
Thanks to the staffs of the Biophysical Collaborative Access Team, a National Institutes of Health-supported Research Center RR08630; the Structural Biology Center Collaborative Access Team, which is supported by U.S. Department of Energy Grant W-31-109-ENG-38; and the Southeast Regional Collaborative Access Team (supporting institutions may be found at www.ser-cat.orgmembers.html) for their assistance in the development of this project. This work was supported by American Heart Association Greater Midwest Affiliate Grant 0435339Z (to J.P.R.O.O.). A.M was supported by a Leverhulme Emeritus Research Fellowship. T.J.W. was supported by Biotechnology and Biological Sciences Research Council Grant BBS_B_09643. Use of the Advanced Photon Source was supported by the U.S. Department of Energy, Office of Science, Office of Basic Energy Sciences, under Contract No. W-31- 109-ENG-38.
Based on an APS press release by Mona Mort.