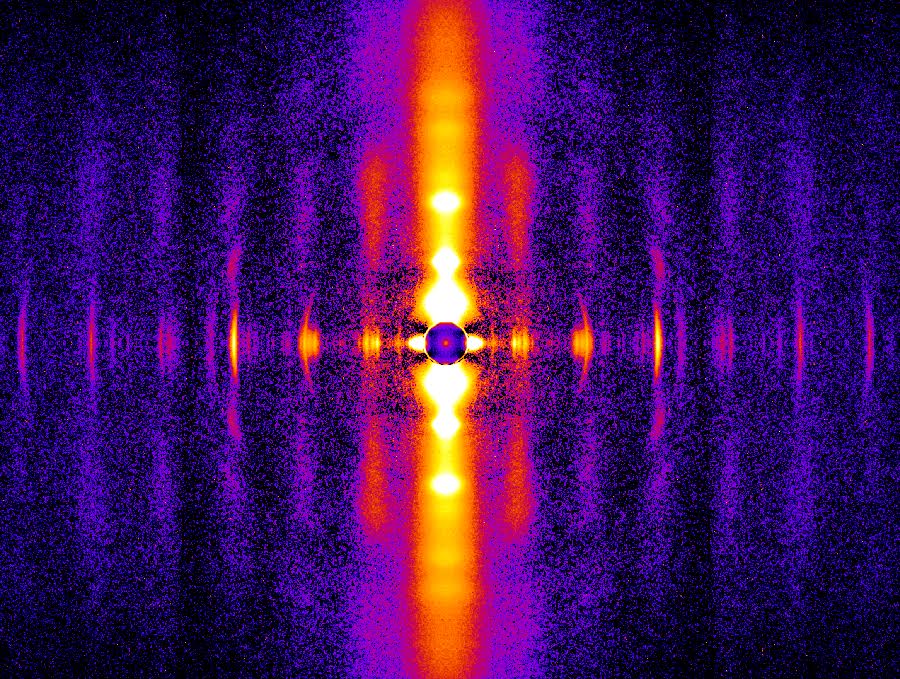
The Frank-Starling law of the heart is a basic physiological principle first observed more than 100 years ago. it describes how the heart is able to move blood through the body in a regulated way by pumping out as much blood as it receives. To understand the nature of the molecular mechanism underlying this important regulatory process, researchers from Loyola University together with colleagues from the Illinois Institute of Technology and the University of Wisconsin–Madison conducted x-ray diffraction experiments at the APS to examine myocardial muscles of rats deficient in length-dependent activation (ldA) of muscle fibers in the heart. Their work shows that the protein titin is critically important for transmitting the stretch-induced signals within the heart’s muscles known to impact the strength with which the heart contracts. This work not only solves a piece of the mystery of how the Frank-Starling law determines cardiac function, but provides an avenue for targeted development of drugs to treat heart failure.
The Frank-Starling law states that the extent to which the heart’s ventricles (and resident muscle fibers) are stretched when filled with blood during the diastolic phase of the cardiac cycle is directly related to the force with which the heart is able to pump blood into the aorta during systole (contraction). This balance is critical for heart function, and is disrupted in patients with heart failure. In these patients, the heart is not able to move the blood that fills the ventricles during diastole through the heart so that it can be sufficiently oxygenated.
Within the walls of the ventricles, muscle fibers are comprised of striated muscle tissue called sarcomeres. Each sarcomere houses contractile proteins arranged into bundles of thin and thick filaments made primarily from proteins myosin and actin, respectively. When these filaments interact, the muscle contracts, generating force. Previous research showed ldA of sarcomeres results from an enhanced response of the contractile proteins to the local concentration of calcium, and that the protein titin, which helps muscles spring back after stretching or contracting, is important for mediating this effect. In fact, mutations in titin are known to disrupt the Frank-Starling mechanism. The nature of titin’s involvement, however, and the reason why muscles, given the same amount of calcium, exert more force when stretched farther, remained unclear.
In this study, the researchers used high-brightness x-rays and the x-ray diffraction technique at the BioCAT x-ray beamline 18-id-d at the APS to observe sarcomere-length induced changes in muscle filaments that harbored either normal (WT) or mutant (hm) titin (fig. 1).
Their results revealed that the length-dependent changes observed in the x-ray diffraction reflections were present only in the normal, WT, muscle. This means that transmission of the “length signal” in the sarcomeres that occurs during filling of the ventricles, depends upon titin to induce the structural alterations in the thick and thin filaments that drive the force of the contraction. Further, these rearrangements correlate with the degree of titin strain, supporting the central role of this protein in the Frank-Starling law.
These findings represent a significant advancement in our understanding of cardiac function and pave the way for future work that may lead to targeted development of therapies for patients with heart failure.
See: Younss Ait-Mou, Karen Hsu, Gerrie P. Farman, Mohit Kumar, Marion L. Greaser, Thomas C. Irving, and Pieter P. de Tombe, “Titin strain contributes to the frank-Starling law of the heart by structural rearrangements of both thin- and thick-filament proteins,” Proc. Natl. Acad. Sci. USA 113(8), 2306 (february 23, 2016). doi: 10.1073/pnas.1516732113
This work was supported, in part, by National Institutes of Health (NIH) grants hl075494 and gm103622. BioCAT is supported by grant 9 P41 gm103622 from the National Institute of General Medical Sciences of the NIH. This research used resources of the Advanced Photon Source, a U.S. Department of Energy (DOE) Office of Science user facility operated for the DOE Office of Science by Argonne National Laboratory under contract no. de-Ac02-06ch11357.
Adapted from an APS press release by Emma Nichols.