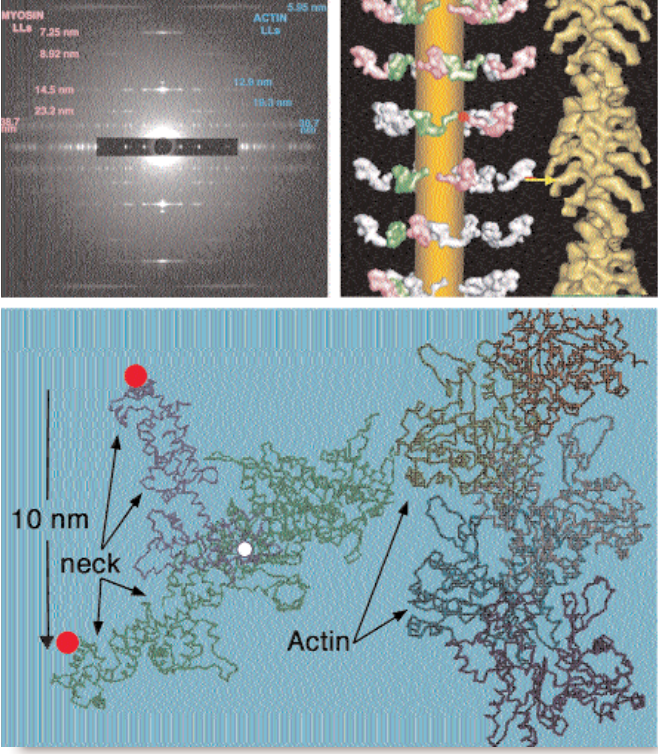
Researchers have achieved the first detailed view of resting muscle filaments poised to contract, a long sought window into the biochemical cycle that causes muscle contraction. The group determined the overall structure of insect muscle fibers from x-ray diffraction patterns and performed computer modeling to analyze the data. The resulting structure, reported in August 2003, suggests a specific mechanism for insect muscle activation.
Muscle cells (fibers) contain two parallel, overlapping sets of protein filaments, made of myosin and actin, respectively, that align with the direction of contraction. The myosin and actin filaments have fixed length individually, but they slide past each other like telescope segments to stretch or shorten the overall muscle. The shortening motion is driven by comma-shaped myosin “heads,” which radiate out from the myosin filaments and cyclically bind to, bend against, and then release adjacent actin filaments. The bind-and-bend phase of each cycle is called a powerstroke. Comparing bound and resting head structures and positions can help researchers deduce the powerstroke mechanism. Researchers had previously viewed the end state of the powerstroke, in which myosin heads have bent and are strongly bound to actin (Fig. 1). No one had reported the orientation of the unbound heads in myosin’s relaxed initial state until August 2003, however.
Researchers from Imperial College London, Duke University, and the Illinois Institute of Technology decided that improved synchrotron technologies and data analysis tools offered the prospect of modeling the relaxed structure. They recorded so-called low-angle x-ray diffraction patterns from flight muscle fibers of giant waterbugs on the BioCAT beamline 18-ID at the APS, which gave them new and sharper details of the large-scale myosin filament structure. A single recording cannot produce both large- and fine-scale data because large structural elements are encoded in low-angle features of the diffraction pattern and vice versa; cameras can resolve one or the other, but not both. So the group computed thousands of different ways of fitting existing high-resolution atomic models of head structure into the overall model, on the basis of the assumption that each head could pivot at two points, and identified the best fit to the observed low-angle x-ray pattern. The model confirmed that resting myosin filaments have stacked rings or “crowns” of eight heads each — two heads per myosin molecule. One head of each myosin projects about 90° from the filament axis; the other tucks inward against the filament’s circumference.
Comparing the results of modeling against actual post-powerstroke myosin head conformations in waterbug and many other species suggests the changes that take place during a powerstroke cycle (Fig. 1). Each of the four projecting heads rotates slightly about the “neck,” which connects to a pivot on the myosin filament. The rotation positions the head to bind tightly to the actin filament and tilt forcefully by 45° relative to the axis of the filament. Such a powerstroke would move the head and bound actin filament 10 nanometers down the axis. The four inward-pointing heads each touch an adjacent projecting head. These contacts may restrain both myosin heads from cleaving high-energy ATP molecules—the powerstroke fuel—until the right moment.
Insect flight muscles must contract rapidly and precisely to keep the insect aloft. As if optimized to do so, the relaxed outward myosin heads seem poised to bind actin and begin powerstroking. The researchers speculate that the increased calcium in stimulated muscle cells may loosen the heads and modify actin filaments to facilitate binding. Stretching flight muscle triggers concerted powerstrokes, perhaps by perturbing the ring of contacts between inward and outward heads to fully enable ATP splitting and actin binding.
See: H.A. Al-Khayat, L. Hudson, M.K. Reedy, T.C. Irving, and J.M. Squire, “Myosin Head Configuration in Relaxed Insect Flight Muscle: X-ray Modeled Resting Cross-Bridges in a Pre-powerstroke State Are Poised for Actin Binding,” Biophys. J. 85, 1063–1079 (August 2003).
J.M.S. was supported from a UK Biotechnology and Biological Sciences Research Council (BBSRC) project grant (28/S10891). M.K.R. was supported by National Institutes of Health AR-14317. CCP13 software was developed as part of UK BBSRC/Engineering and Physical Sciences Research Council (EPSRC) funded projects (e.g., 28/B10368 and 28/B15281). Use of the Advanced Photon Source was supported by the U.S. Department of Energy, Basic Energy Sciences, Office of Energy Research, under Contract No. W-31-109-Eng-38. BioCAT is a U.S. National Institutes of Health-supported Research Center RR08630.