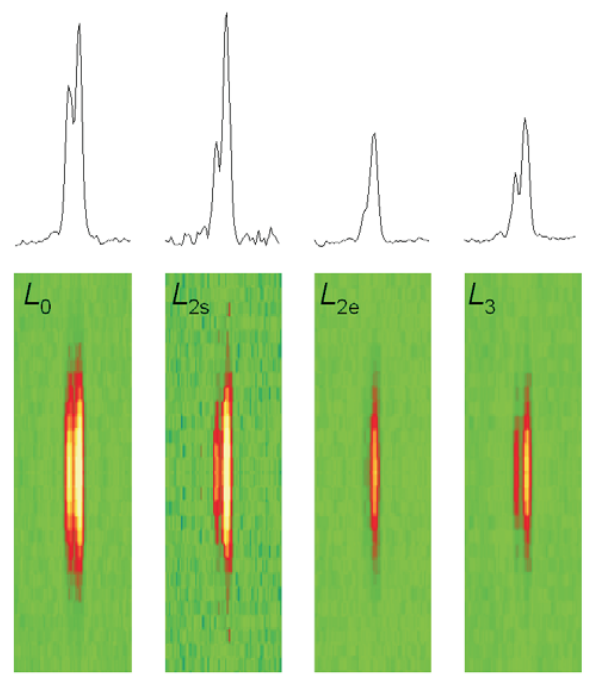
The sliding filament model of muscle contraction is more than 50 years old, yet theories about the precise mechanisms of the motor function still generate some controversy. A group of researchers from Università di Firenze, Istituto Nazionale di Fisica della Materia, Brandeis University, King’s College London, the European Synchrotron Radiation Facility, Illinois Institute of Technology, and Argonne National Laboratory used insertion device beamlines at BioCAT at the APS and at the European Synchrotron Radiation Facility (ESRF) to take a closer look at the molecular structure of myosin II, the molecular motor in muscle, as it works under different loads. This study provides a more detailed understanding of the complex process responsible for the efficient chemomechanical transduction of energy.
Myosin II is a class of proteins found in skeletal muscle and heart cells. Myosin molecules aggregate to form the thick myosin filament, with the heads of the molecules protruding periodically from the filament. Myosin filaments overlap with the actin filaments in the 2.1-μm sarcomere, the elementary contracting structure in muscle. During contraction, the myosin heads act as tiny motors, drawing the thin actin filament toward the center of the sarcomere and then detaching from actin and returning to the original conformation, ready for another stroke cycle.
Studies using in vitro samples of muscle tissue have produced variable results in measuring the size of the stroke produced by the intermittent binding of myosin to actin. These puzzling results are likely attributable to the nature of the samples, which do not retain their natural structure and function and thus are inaccurate measurements of events in a muscle fiber in actual physiological conditions.
To produce a more precise view of these molecular interactions, researchers have recently used an x-ray diffraction technique to measure the size of the working stroke in vivo, by using intact frog skeletal muscle cells. The researchers directed parallel synchrotron x-ray beams to the muscle cell. This produces a strong reflection called M3, which comes from the 14.5-nm repeat of the myosin heads along the filament (Fig. 1). The x-ray interference originating from the two arrays of myosin heads in each sarcomere allows measurements of the axial motion of the motors with ~1 Å spatial resolution and submillisecond time resolution.
At the 18-ID (APS) and ID-2 (ESRF) beamlines, the researchers measured changes in intensity and in the fine structure of the reflection during three phases of the motor cycle elicited at three different loads: 0.25, 0.50, and 0.75 times the isometric force (T0). To eliminate the damaging effect of radiation, which eventually caused the fibers to fail, the samples were displaced vertically a few hundred micrometers between each contraction. A steady isometric force was obtained by electric stimulation of the fiber at the proper frequency—ranging from 18 to 25 Hz at the 4°C used in these experiments. Then the force was suddenly reduced in a stepwise manner through a force feedback control. During the filament sliding that follows this step, the two interference peaks into which the M3 x-ray reflection is split change their relative intensities. First R, the ratio of the high-angle peak over the low angle peak, decreases; then R increases as the myosin heads detach and return to their original axial position.
The recovery of R occurs at ~6 nm with 0.75 T0 and at ~11 nm with 0.25 T0, showing that the axial motion that drives filament sliding is smaller at high loads.
These observations help explain the efficiency of muscle fibers at the molecular level.
See: M. Reconditi, M. Linari, L. Lucii, A. Stewart, Y.-B. Sun, P. Boesecke, T. Narayanan, R.F. Fischetti, T. Irving, G. Piazzesi, M. Irving, V. Lombardi, “The Myosin Motor in Muscle Generates a Smaller and Slower Working Stroke at Higher Load,”” Nature 428(6982), 578 (1 April 2004).
This work was supported by Ministero dell’Istruzione, dell’Università e della Ricerca, Telethon-945 (Italy), the National Institutes of Health (NIH, USA), the Medical Research Council (UK), the European Molecular Biology Laboratory, the European Union, and the European Synchrotron Radiation Facility. Bio-CAT is an NIH-supported research center. Use of the Advanced Photon Source was supported by the U.S. Department of Energy, Office of Science, Office of Basic Energy Sciences, under Contract No.W-31-109-ENG-38.
Based on APS press release by Elise LeQuire.